Raman spectroscopy uses intense light from a laser to probe the chemical bonds in a substance, generating a spectrum that acts as a fingerprint which can be used to characterize or identify the substance. This can be used to authenticate materials or assess their quality, and even for medical diagnostics. But how does a Raman spectrometer work?
A Raman spectrometer system requires three primary components:
- A high-intensity laser
- A sample interface
- A spectrometer
The laser acts as the excitation source which provides the high intensity light required to obtain sufficient Raman signals for detection. Raman scattering occurs at a probability of one part in a million, so high laser intensities are required to balance out the rarity of Raman scatter. Although any wavelength can be used to generate a Raman spectrum, the excitation wavelength is the defining factor underlying Raman intensity.
Scattering intensity is inversely proportional with the excitation wavelength to the fourth power (λ-4), which means shorter laser wavelengths yield a greater Raman signal and vice versa. Therefore, an ultraviolet Raman laser can obtain a spectrum which is orders of magnitude more intense than that of a near-infrared laser. Conversely, shorter wavelengths are more likely to induce autofluorescence than longer ones, which will obscure the Raman signal. Selecting the right laser wavelength subsequently depends primarily on sample type, balancing signal intensity against autofluorescence for the material under investigation.
Read More: How Choice of Excitation Wavelength Affects Your Raman Spectrum
The Raman spectrometer system’s sample interface refers to the optics which direct and focus the incident beam, and which collect the comparatively weak Raman emissions for routing to the spectrometer itself. A longpass dichroic filter is commonly used to reflect shortwave laser light onto the sample and to transmit Raman scattered light through to the spectrometer, as shown below. An additional longpass filter with a minimum optical density (OD) of 6 is typically integrated into the sampling optics to block the overwhelming Rayleigh scatter from the excitation laser light. Sampling optics for Raman systems come in various optical configurations: some use fiber optic coupling to allow the sampling optics to be integrated into a probe for use at a distance from the spectrometer, while others use fully integrated sampling optics to reduce size and optical losses within the system.
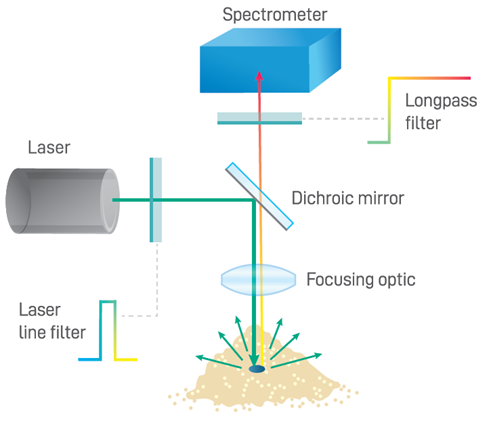
Lastly, the Raman spectrometer simultaneously captures and detects all the light transmitted by the sample interface, reporting a spectrum as a function of Raman shift relative to laser frequency. This requires sufficient range, signal strength, and optical resolution. Key performance properties include high sensitivity, a good signal-to-noise ratio, and a high light collection power, expressed as the f-number (f/#) or numerical aperture (NA). Spectrometer range is another important parameter to consider. This refers to the Raman shift frequency range, which is typically broken up into the fingerprint region (<1500 cm-1), used for material identification, and the functional region (<3600 cm-1), which provides additional chemical bond information used in research and specialized applications.
Interested in Raman spectrometers? Refer to our Raman spectroscopy product page for more information on modular, semi-integrated, and fully-integrated Raman systems.