Finding the right matrix for pH
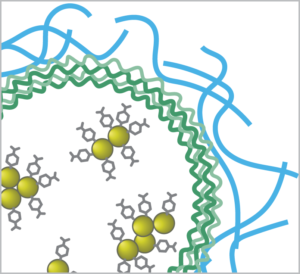
Implantable biosensors herald hope as an accurate, continuous, and proactive non-invasive monitoring method for people living with chronic metabolic conditions like diabetes, to eliminate the distress and inconvenience of regular blood sampling. The technology may also someday benefit the healthy, or those at risk for certain conditions. By tracking long-term trends within a specific individual, it may be possible to detect early signs of disease that would otherwise be missed until serious symptoms arise.
These “smart” sensing implants are the focus for Mike McShane’s group at Texas A&M University’s Department of Biomedical Engineering and Center for Remote Health Technologies & Systems. Surface enhanced Raman spectroscopy (SERS) is one of the optical technologies they are exploring for this purpose through the work of PhD student Dayle Kotturi1. Their unique approach involves incorporating gold nanoparticle-based assays into capsules made of polyelectrolyte multilayer (PEMs), and stabilizing these sensors in a hydrogel material that could someday be implanted in the body and read non-invasively with a handheld Raman spectrometer.
Download the App NoteWhy Raman for Biosensors?
The ideal implantable biosensor must be sensitive and specific, even in the presence of complex bodily fluids. It must also be biocompatible and stable over long periods so as to deliver consistent outputs and minimize the frequency of sensor replacement.
Although Raman spectroscopy is both highly selective and non-invasive, it yields low signal levels that have traditionally required the use of benchtop systems for detection. As a result, it has not yet seen the same success as fluorescence-based sensors like the glucose monitor now approved by the FDA for in-vivo use2. By using plasmonic nanoparticles to leverage the signal enhancement offered by SERS, however, Raman becomes a highly sensitive, non-invasive technique that can be performed with handheld instruments. When applied in this way, SERS offers several advantages over fluorescence for biosensors.
Fluorescence-based sensors are prone to photobleaching, and thus offer a limited lifetime – an undesirable drawback when the intended application is in-vivo and long-term. SERS, in contrast, offers equal or greater sensitivity at lower laser power, and as they are not susceptible to photo-bleaching, SERS sensors could offer a much longer operational lifetime.
The peaks in Raman are narrow, and thus are more easily separated and assigned than broad fluorescence peaks. The unique Raman fingerprint carried by each molecule that makes the technique highly selective also makes it ideal for multiplexing. By using different SERS sensor molecules for each target analyte and deconvoluting their relative contribution in software, multiple biological parameters could be tracked simultaneously.
BIOSENSOR TECHNOLOGY | Fluorescence | Raman/SERS |
Status | Established technique | Becoming viable |
Detection method | Single band: Excitation LED/laser & filter/photodiode | Multi-band: Excitation laser & broadband spectrometer |
Size | Small, all sensing elements can fit into a small plastic package | Benchtop, but getting smaller and cheaper with handheld systems |
Peak widths | Wide, overlapping | Narrow, specific |
Photobleaching | Susceptible, limits lifetime | Not susceptible |
Multiplexing potential | Limited | High |
The Making of a SERS-Based Biosensor
The biosensor approach developed by McShane’s TAMU group begins with gold nanoparticles (AuNP) – plasmonic nanoparticles that enhance the Raman signal. These are functionalized with a Raman probe specific to the analyte of interest, and then enclosed in polyelectrolyte multilayers (PEM) to create capsules.
This innovative sensor design allows small molecule analytes to pass into the capsules for detection, while the larger biomolecules that often interfere with biosensor response are rejected. Choice of the hydrogel material used to stabilize the capsules is key to biocompatibility, sensitivity, and longevity in-vivo.
Having demonstrated the sensitivity and reproducibility of the SERS probes embedded in a hydrogel matrix in previous work3,4, the next step was to test the viability of the SERS biosensor in a simulated in-vivo environment, with pH as a good general analyte of interest. Gold nanoparticles conjugated with MBA (4-mercaptobenzoic acid) were used to create the pH-sensitive SERS assay. Three types of biocompatible hydrogel matrix materials were then embedded with the pH sensor capsules for testing, each selected for their minimal Raman response and promising biocompatibility:
-
- Alginate (used in previous work)
- PEG: polyethylene glycol
- pHEMA: poly(2-hydroxyethyl methacrylate)
Sensitive Sensing
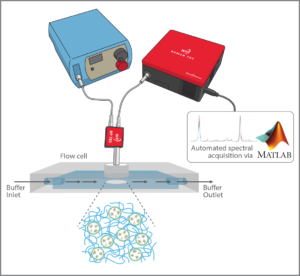
The high sensitivity of the Wasatch Photonics system allowed very little laser power to be used – less than 20 mW – minimizing potential tissue damage while still achieving good signal at a 5 second integration time (ample given the slow rate of change of metabolic parameters within the body). The system yielded consistent peak reproducibility over time and over the wavelength spectrum, both of which are essential to creating a dependable biosensor.
A flow system pumped liquid through the flow cell at the gradual rate of 4 mL/min, taking spectra every 2 minutes using an automated data collection program utilizing our MATLAB® software driver. This enabled the experiment to be run continuously for 48-60 hours unattended, with dependable spectral output. Future work will utilize our LabVIEW driver to enable the standalone laser to be triggered only when needed, as opposed to running continuously. The system as designed allowed the sensitivity, accuracy, repeatability and reversibility of the pH-sensitive SERS sensors in each hydrogel matrix to be evaluated, putting them through their paces at pH 4, 7, and 10.
Selectivity: pH Response Discrimination
The Raman spectrum of MBA features two pH-sensitive peaks, one which scales proportionally with pH at 1430 cm-1, and the other inversely with pH at 1702 cm-1. These lie in the shadows of a much stronger pH-insensitive peak at 1582 cm-1, which was used as a reference to scale the response of the pH peaks.
The impact of hydrogel matrix on sensor specificity was assessed by looking at the Raman spectra for each in three pH buffers: 4, 7, and 10. The three hydrogels varied in their ability to distinguish between the pH levels, with pHEMA offering the clearest distinction between the pH levels at both pH-sensitive peaks of MBA. Alginate could distinguish between all three pH levels clearly at the 1430 cm-1 peak, but registered pH 7 and 10 very similarly at 1702 cm-1. PEG was able to distinguish only between pH 4 and higher pH values, but not between pH 7 and 10, ruling it out as a possible hydrogel matrix.

Stability: pH Response Repeatability
The impact of hydrogel matrix on sensor stability and reversibility was assessed using continuous flow studies, cycling through the three pH levels randomly over a period of 24 hours and giving the sensor at least 2 hours to come to steady state before changing pH. Automation of this process through software allowed spectra to be collected for long periods without supervision.
In this test, alginate shone, showing its signal level to be repeatable, reversible, and stable for both SERS peaks as pH level was changed. PEG showed signal drift over time for pH 4, but was more repeatable at pH 7 and 10. In contrast, pHEMA was repeatable for pH 4, but was slower and showed drift in signal in response to changes to pH 7 and 10.
Thus although pHEMA seemed more specific to each individual pH, alginate yielded much more repeatable results that would allow each pH to be confidently identified based on signal level in a continuously variable pH environment. The downside of using alginate is that it must be stabilized using CaCl2 in the buffer, which wouldn’t be possible in the human body.
“Sense-able” Answers
This in-vivo simulation study of SERS-active pH sensing assays demonstrated the potential of the technology for implantable biosensors. It showed how the choice of hydrogel matrix can impact selectivity, repeatability, and reversibility, highlighting its role in stabilizing the pH sensor capsules to optimize biocompatibility & longevity. By looking at just three possible hydrogel matrix materials, it was possible to demonstrate both excellent selectivity for pH and repeatable sensing throughout nine pH changes over an extended period of time.
There are thousands more possible media to explore, and the opportunities are great to find an ideal hydrogel matrix that meets the SERS biosensors’ needs from a detection aspect while offering good biocompatibility. With a highly sensitive, automated flow and detection system, and a well-designed microcapsule sensor assay, the TAMU group is well-equipped to do just that. And as they create new biosensor assays for this and other metabolic parameters, we’ll be designing ever-smaller, more sensitive Raman instruments to take them into the field.
References
- Kotturi, Dayle, et al. “Evaluating hydrogels for implantable probes using SERS.” Plasmonics in Biology and Medicine XVI. Vol. 10894. International Society for Optics and Photonics, 2019.
- Commissioner, Office of the. “FDA Approves First Continuous Glucose Monitoring System with a Fully Implantable Glucose Sensor and Compatible Mobile App for Adults with Diabetes.” U.S. Food and Drug Administration, FDA, 21 June 2018, www.fda.gov/news-events/press-announcements/fda-approves-first-continuous-glucose-monitoring-system-fully-implantable-glucose-sensor-and.
- You, Yil-Hwan, et al. “SERS-based hydrogel sensors for pH and enzymatic substrates.” 2015 IEEE SENSORS. IEEE, 2015.
- You, Yil-Hwan, et al. “SERS-active Smart Hydrogels with Modular Microdomains: from pH to glucose sensing.” IEEE Sensors Journal 17.4 (2017): 941-950.